EM studies have shown that nucleus consist of,
1.Nuclear Envelope
2.Nuclear Matrix
3.Nucleoplasm
4.Nucleolus
5.Chromatin
1.Nuclear Envelope:
Transmission electron microscopy revealed that the nucleus is bounded by a nuclear envelope composed of two membranes the inner and outer nuclear membranes separated by a perinuclear space measuring about 20–40 nm across. The outer nuclear membrane is continuous with the endoplasmic reticulum, making the perinuclear space continuous with the lumen of the ER. Like membranes of the rough ER, the outer membrane is often studded on its outer surface with ribosomes engaged in protein synthesis. Several proteins in the outer membrane bind to actin and intermediate filaments of the cell’s cytoskeleton, thereby anchoring the nucleus within the cell and providing a mechanism for nuclear movements.
Tubular invaginations of the nuclear envelope, collectively known as the nucleoplasmic reticulum, may project into the internal nuclear space and increase the area of the nucleus that makes direct contact with the inner nuclear membrane. One of the most distinctive features of the nuclear envelope is the presence of specialized channels called Nuclear pores, which are especially easy to see when the nuclear envelope is examined by freeze-fracture microscopy. Each pore is a small cylindrical channel extending through both membranes of the nuclear envelope, thereby providing direct continuity between the cytosol and the nucleoplasm. The number of pores varies greatly with cell type and activity. A typical mammalian nucleus has about 3000–4000 pores, or about 10–20 pores per square micrometer of membrane surface area.
At each pore, the inner and outer membranes of the nuclear envelope are fused together, forming a channel that is lined with an intricate protein structure called the nuclear pore complex (NPC). The NPC has an outer diameter of 120 nm and is built from about 30 different proteins called nucleoporins. In electron micrographs, the most striking feature of the pore complex is its octagonal symmetry. It is made up of rings of eight subunits arranged in an octagonal pattern. In other views, the eight subunits are seen to protrude on both the cytoplasmic and nucleoplasmic sides of the envelope. Nuclear pore complex regulate the transport of solutes in between cytoplasm and nucleoplasm, it also participate in maturation of Ribosomes.
2. Nuclear Matrix
In the early 1970s researchers discovered that an insoluble fibrous network retaining the overall shape of the nucleus remains behind after more than 95% of the chromatin has been removed by a combination of nuclease and detergent treatments. This network, called the nuclear matrix or nucleoskeleton, is thought to help maintain the shape of the nucleus and provide an organizing skeleton for the chromatin fibers. Underlying the inner nuclear membrane is the nuclear lamina, a filamentous meshwork that provides structural support to the nucleus. The nuclear lamina is composed of 60- to 80-kilodalton (kd) fibrous proteins called lamins, along with associated proteins. Nucleolamins help in stability of nuclear membrane and chromatin.
3. Nucleoplasm: (Strasburger, 1882). It is a transparent, semi-fluid and colloidal substance which fills the nucleus. It contains nucleosides and a number of enzymes (e.g., DNA polymerase, RNA polymerase, nucleoside phosphorylase) which are required for the synthesis and functioning of DNA, RNA, nucleoproteins, etc. Some of the proteins present in nucleoplasm are essential for spindle formation.
4. Nucleolus: It was first discovered by Fontana in 1781, described by Wagner in 1840 and provided with its present name by Bowman in 1840. Nucleolus is a naked, round or slightly irregular structure which is attached to the chromatin at a specific region called Nuclear Organiser Region (NOR). Commonly 1-4 nucleoli are found in a nucleus. Up to 1600 nucleoli are reported in the oocytes of Xenopus. A covering membrane is absent around nucleolus. Calcium seems to be essential for maintaining its configuration. Nucleolus has four components-
⁕Amorphous matrix,
⁕Granular part,
⁕Fibrillate portion and
⁕Chromatin part
(a) Amorphous Matrix. It is the homogeneous ground substance of the nucleolus. Matrix is formed of protein.
(b) Granular Portion. It consists of granules of the size of 150-200 Å which lie scattered in the amorphous matrix. The granules are formed of protein and RNA in the ratio of 2 : 1. They are believed to be precursors of ribosomes.
(c) Fibrillar Portion (Nucleolonema). It is formed of a large number of small fibrils that are 50-80 Å long. The fibrils are made up of both protein and RNA and are believed to be precursors of granules.
(d) Chromatin Portion. It is that part of chromatin which is associated with nucleolus. Depending upon its position nucleolar chromatin is of two types- perinucleolar and intranucleolar. The perinucleolar chromatin lies around the periphery of the nucleolus. It gives rise to in growths or trabeculae which produce the intranucleolar chromatin.
The nucleolus is the site for ribosomal RNA synthesis. The nucleoli are larger and more numerous in cells that are actively involved in protein synthesis. Isolated nucleoli contain the nucleolus organizer region (NOR) -a stretch of DNA carrying multiple copies of rRNA genes. These multiple rRNA genes occur in all genomes and are thus an important example of repeated DNA that carries genetic information. The number of copies of the rRNA genes varies greatly from species to species, but animal cells generally contain hundreds of copies and plant cells often contain thousands. The multiple copies are grouped into one or more NORs, which may reside on more than one chromosome; in each NOR, the multiple gene copies are tandemly arranged. They are also called secondary constriction.
In humans secondary constriction is present in chromosome no. 13,14,15, 21 & 22.
Chromatin: It is hereditary DNA-protein fibrillate complex which is named so because of its ability to get stained with certain basic dyes (Gk. chroma- colour, Flemming, 1879). Chromatin occurs in the form of fine overlapping and coiled fibers which appear to produce a network called chromatin reticulum. Chromatin fibers are distributed throughout the nucleoplasm. They are differentiated into two regions- Euchromatin and Heterochromatin. Euchromatin is narrow (30-80 Å thick) lightly stained and diffused fibrous part which forms the bulk of chromatin. Heterochromatin is wider (250 Å thick), darkly stained and condensed granular part which is attached here and there on the euchromatin. Depending upon the size of granules formed by heterochromatin they are called chromocentres, karyosomes or false nucleoli.
Euchromatin
Euchromatin is a lightly packed form of chromatin (DNA, RNA, and protein) that is enriched in genes, and is often (but not always) under active transcription. Euchromatin comprises the most active portion of the genome within the cell nucleus, 92% of the human genome is euchromatic.
It enters into replication first and lightly stained. The basic structure of euchromatin is an elongated, open, 10 nm microfibril. It has a beads on a string structure or Nucleosome structure. Nucleosomes were first observed as particles in the electron microscope by Don and Ada Olins in 1974, and their existence and structure (as histone octamers surrounded by approximately 200 base pairs of DNA) were proposed by Roger Kornberg.
In 1974, using the data from nuclease digestion and other types of information, Roger Kornberg, proposed that DNA and histones are organized into repeating subunits, called nucleosomes. Nucleosome is formed by the octamer of histone proteins, around this octamer 200 bps of DNA is wrapped. The formation of nucleosomes converts a DNA molecule into a chromatin thread that is approximately one-third the length of the initial piece of DNA, and it provides the first level of DNA packing.
All four of the histones that make up the octamer are relatively small proteins, with a high proportion of positively charged amino acids (lysine and arginine). The positive charges help the histones bind tightly to the negatively charged sugar phosphate backbone of DNA. These numerous electrostatic interactions explain in part why DNA of virtually any sequence can bind to a histone octamer. Each individual nucleosome core particle consists of a complex of eight histone proteins-two molecules each of histones H2A, H2B, H3, and H4-and double-stranded DNA that is 147 nucleotide pairs long. The histone octamer forms a protein core around which the double-stranded DNA is wound. Each nucleosome core particle is separated from the next by a region of linker DNA, which can vary in length from a few nucleotide pairs up to about 80. On average, therefore, nucleosomes repeat at intervals of about 200 nucleotide pairs. For example, a diploid human cell with 6.4 x 109 nucleotide pairs contains approximately 30 million nucleosomes.
In interphase nucleus generally chromatin exists in two forms Euchromatin and Heterochromatin. Usually Euchromatin exists in Beads on a string form while Heterochromatin are at higher condensation level i.e. 30 nm chromatin fiber which is the Solenoid form which is formed with the help of H1 histone protein.
Chromosomes is the condensed form of chromatin visible during dividing or M-Phasee. The chromosomes are capable of self-reproduction and maintaining morphological and physiological properties through successive generations. They are capable of transmitting the contained hereditary material to the next generation. Hence these are known as ‘Hereditary vehicles.
•Chromosomes were first observed by Karl Negali in 1842 in plant cells as rod-shaped bodies in plant cells.
•In 1848 Hofmeister Observed chromosomes as darkly stained bodies in pollen mother cells of Tradecscantia.
•In 1888 Waldayer coined the term chromosome.
•In 1902 Walter S. Sutton proposed ‘Theory of Chromosomal basis of Heredity.
The salient features of chromosome theory of heredity are: -
•The somatic cells of an organism which are derived by the repeated divisions of the zygote are all diploid, i.e. these consist of two identical sets of chromosomes. One set of chromosomes is received from mother (maternal set) through ovum and the other set is inherited from father through sperm (paternal set). These two chromosomes of one type constitute the homologous pair.
•The chromosomes retain their structural uniqueness, individuality and their continuity throughout the life-cycle of an organism.
•Each chromosome carries specific determiners or Mendelian factors and plays a significant role in the development of an organism from the zygote.
•The behavior of chromosomes during meiosis, which occurs at the time of gamete formation provides an evidence that genes or determiners are located in the chromosomes. This also explains the mechanism of segregation of characteristics at the time of gamete formation.
Number of Chromosomes:
The number of chromosomes in the somatic cells of higher animals and plants is known as diploid or somatic or zygotic number, while in the gametes (sperms and eggs) it is haploid, gametic or reduced. The number of chromosomes is constant in all the somatic cells of all the individuals of a species. Chromosome number is used in the identification of species and in tracing the relationship within the species.
The lowest no of chromosomes are present in,
Parascaris = No of chromosomes are 2,
Mucor hiemalis = No of chromosomes are 2,
Happlopappus gracilis = No of chromosomes 2n = 4
While highest no of chromosomes are present in,
In plants it is Adder’s Tongue fern
Ophioglossum retiulatum = 2n = 1262 while in animals,
Aulocantha = 2n = 1632
CHROMOSOME CYCLE AND CELL CYCLE
Chromosomes exhibit cyclic change in shape and size during cell cycle. In the non dividing interphase nucleus, the chromosomes form an interwoven network of line twisted but uncoiled threads of chromatin, and are invisible. During cell division the chromatin threads condense into compact structures by helical coiling. In prophase of cell division the chromosomes appear as distinct threads and by metaphase and also in anaphase these become short, compact bodies having definite shapes and sizes. In anaphase these appear as rod-shaped, V-shaped, L-shaped or J-shaped. The chromosomes are studied and described at this stage or at anaphase stage. In telophase these again uncoil to form the chromatin net.
Chromosomes differ greatly in size in different organisms. Even the chromosomes of different pairs in the nucleus of the same cell have different sizes. The anaphase chromosomes range from 1 μm to 30 μm in length and 0.22 μ to 2 μ in width.
A chromosome consists of following parts,
⁕Centromere
⁕Telomere
⁕Secondary Constriction,
Centromere:
Centromere is the part of chromosome where two chromatids join with each other. At centromere specific proteins bind and form a disc like structure called Kinetochore, at kinetochore spindle fibers interact and help in the separation of sister chromatids (Chromatids which join with same centromere in a chromosome are called Sister Chromatids).
The centromeric region is also called Primary constriction. The microtubules of the chromosomal spindle fibers are attached to the centromere. Therefore, centromere is associated with the chromosomal movement during cell division. A chromosome without centromere is lost during anaphase.
Chromosomes are classified by two ways on the basis of centromeres,
1.On the basis of number of centromeres
2.On the basis of position of centromeres
On the basis of number of centromeres:
Usually, there are two centromeres one on each chromatid but these may be more or none. Depending upon the number of centromeres, the chromosomes may be:
1.Monocentric with one centromere.
2.Dicentric with two centromeres, one in each chromatid.
3.Polycentric with more than two centromeres.
4.Acentric without centromere. Such chromosomes represent freshly broken segments of chromosomes which do not survive for long.
5.Diffused or non-located with indistinct centromere diffused throughout the length of chromosome. The microtubules of spindle fibers are attached to chromosome arms at many points. The diffused centromeres are found in insects, some algae and some groups of plants (e.g. Luzula).
2.On the basis of position of centromeres:
Based on the location of centromere the chromosomes are of four types,
1.Acrocentric are rod-shaped chromosomes with centromere occupy terminal position. These chromosomes appear ‘i’ shaped during anaphase.
2.Telocentric are rod-shaped chromosomes having subterminal centromere. These chromosomes appear ‘j’ shaped during anaphase.
3.Submetacentric are S-shaped chromosomes with centromere slightly away from the mid-point so that the two arms are unequal. These chromosomes appear ‘L’ shaped during anaphase.
4.Metacentric are large chromosomes in which centromere lies in the middle of chromosome so that the two arms are almost equal. These chromosomes appear ‘V’ shaped during anaphase.
5.
Telomere:
Telomere constitute the end of chromosomes. The number of telomere varies in between 2 to 4. Hermann J. Muller, who introduced the term telomere in 1938, demonstrated that Drosophila chromosomes without natural ends—produced by breaking chromosomes with X rays—were not transmitted to progeny. In a classical study of maize chromosomes, Barbara McClintock demonstrated that new ends of broken chromosomes are sticky and tend to fuse with each other. In contrast, the natural ends of normal (unbroken) chromosomes are stable and show no tendency to fuse with other broken or native ends. McClintock’s results indicated that telomeres must have special structures different from the ends produced by breakage of chromosomes. The telomeres of eukaryotic chromosomes have unique structures that include short nucleotide sequences present as tandem repeats. Although the sequences vary somewhat in different species, the basic repeat unit has the pattern 5’ T1–4A0–1G1–8-3’ in all but a few species. For example, the repeat sequence in humans and other vertebrates is TTAGGG, that of the protozoan Tetrahymena thermophila is TTGGGG, and that of the plant Arabidopsis thaliana is TTTAGGG. In most species, additional repetitive DNA sequences are present adjacent to telomeres; these are referred to as telomere-associated sequences.
These Telomere associated sequences can roll back on similar sequences and close the free end of the chromatids. This structure is also stabilized with the help of certain proteins. The telomeres of humans and a few other species have been shown to form structures called t-loops, in which the single strand at the 3’ terminus invades an upstream telomeric repeat (TTAGGG in mammals) and pairs with the complementary strand, displacing the equivalent strand. The DNA in these t-loops is protected from degradation and/or modification by DNA repair processes by a telomere-specific protein complex called Shelterin.
The telomeric sequences are under constant attack by exonucleases and maintained by continuous synthesis with the help of a unique enzyme Telomerase.
Secondary Constriction or Nucleolar Organizer
Sometimes one or both the arms of a chromosome are marked by a constriction other than the primary constriction. During interpose this area is associated with the :nucleolus and is found to participate in the formation of necleolus. It is, therefore, known as Nucleolar Organizer Region or the Secondary constriction. its location on the chromosome is marked with a lightly stained constricted region.
Nucleolar Organizer Region (NOR):
In certain chromosomes, the secondary constriction is intimately associated with the nucleolus during interphase. It contains genes coding for 18S and 28S ribosomal RNA and is responsible for the formation of necleolus. It is, therefore, known as nucleolar organizer region (NOR). Its location in the chromosome is marked by a lightly stained constricted area and is constant for a given chromosome. The terminal part of a chromosome beyond secondary constriction is called Satellite.
A karyotype is an individual's collection of chromosomes. The term also refers to a laboratory technique that produces an image of an individual's chromosomes. The technique to generate karyotype is called Karyotyping.
Karyotypes describe the chromosome count of an organism and what these chromosomes look like under a light microscope. Attention is paid to their length, the position of the centromeres, banding pattern, any differences between the sex chromosomes, and any other physical characteristics.
The preparation and study of karyotypes is part of cytogenetics. In 1955 Joe Hin Tjio working in Albert Levan's developed Human Karyotype. In human karyotype there are 23 pairs of chromosomes in which 22 pairs are called Autosomes while one pair determine the sex and called Allosome.
A karyotype may include the number of chromosomes, relative size, position of centromere, length of the arm, secondary constriction and satellites, features by which a particular set of chromosome can be arranged. A diagrammatic representation of karyotype (morphological characteristic of the chromosomes) of a species is called Idiogram, where the chromosomes of an organism are ordered in a series of decreasing size.
Depending upon complexity Karyotype is of two types,
Identification of chromosomes and karyotyping can be done by following techniques:
Chromosome Banding:
Chromosome banding is the “Lengthwise variation in staining properties along a chromosome”. Chromosome banding refers to alternating light and dark regions along the length of a chromosome, produced after staining with a dye. A band is defined as the part of a chromosome that is clearly distinguishable from its adjacent segments by appearing darker or lighter with the use of one or more banding techniques. Torbjorn Caspersson when working with plants and using the highly fluorescent quinacrine mustard produced the first chromosome banding patterns, which were reported in 1968. The alternating bands of bright and dull fluorescence were called Q-bands. Quinacrine-bright bands were composed primarily of DNA rich in the bases adenine and thymine. Quinacrine mustard staining was applied to human chromosomes to produce the first-banded human karyogram in 1970. Analyses of Q-banded chromosomes led to the unequivocal identification of each human chromosome, and within each chromosome, specific regions and bands, since then variety of banding techniques are developed.
Classification of Banding Techniques
Based on
•GC and AT rich regions.
•Constitutive Heterochromatin Region.
Banding is always carried out in metaphase chromosomes whose size has condensed and whose diameter is increased are used for chromosome banding studies after fixing the stage.
Banding Techniques
Besides Banding many new and powerful techniques are developed such as Fluorescence in situ hybridization (FISH) and multicolour fluorescence in situ hybridization (McFISH) which have been extensively used in animals and plants. Here using DNA probes, labelled with radioactive or nonradioactive molecules are used to locate the positions of specific DNA sequences on chromosomes. In McFISH, DNA may also be labelled with fluorochromes to allow the use of one or more colors to locate the position of one or more DNA sequences simultaneously on the same chromosomes.
Both FISH and McFISH are highly specific and are widely used in Medical Diagnosis, Evolutionary and population studies.
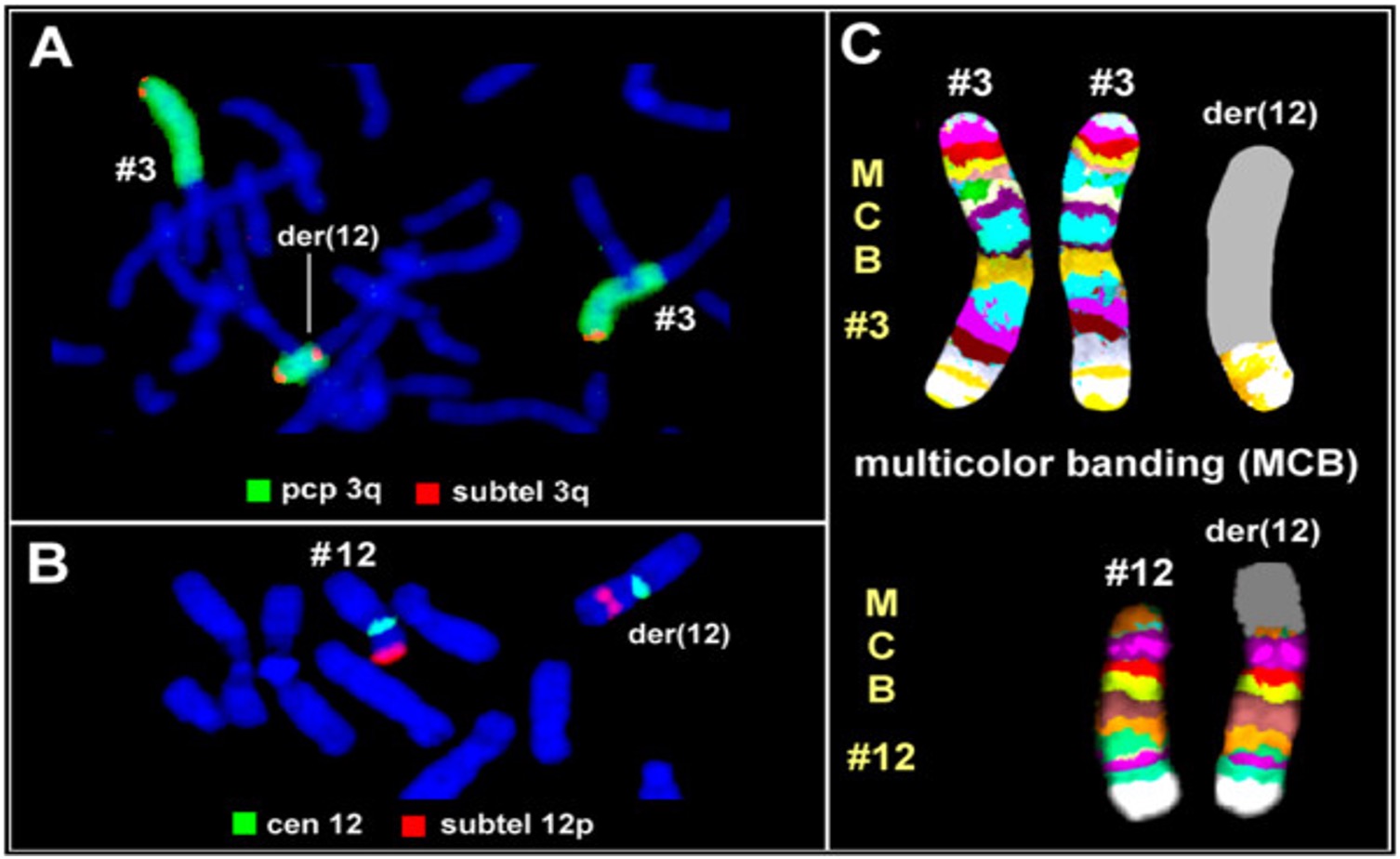
Lampbrush chromosomes
Lampbrush chromosomes were discovered in salamander egg cells (Ambystoma mexicanum) by Flemming in 1882. Later on they were observed by Rückert in 1882 who introduced the term “Lampbrush chromosome” into biological nomenclature. The chromosomes obtain their name from the 19th century brushes that were used for cleaning street lamps. Lampbrush chromosomes (LBCs) are transcriptionally active chromosomes found in the germinal vesicle (GV) of large oocytes of many vertebrate and invertebrate animals and also in the giant single-celled alga Acetabularia. Lampbrush chromosomes are meiotic arrested homologous pair of chromosomes which are enlarged for yolk formation.
Size:
Lampbrush chromosomes are intermediate structures present during the first meiotic division. In a prolonged diplotene stage, they undergo decondensation that results in the production of very large chromosomal structures. LBCs’ length ranges (depending on the species) from 400 to 800 mm, which makes them up to 30 times larger than their mitotic counterparts.
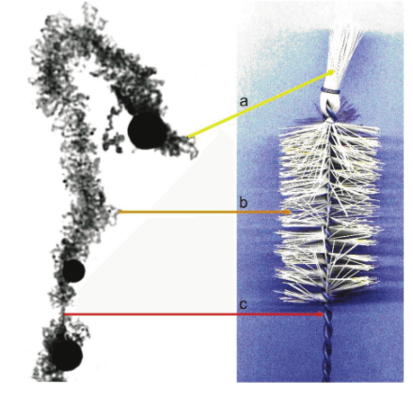
Large chromosomes
Chromosomes transform into the lampbrush form during the diplotene stage of meiotic prophase I due to an active transcription of many genes. They are highly extended meiotic half-bivalents, each consisting of 2 sister chromatids. Lampbrush chromosomes are clearly visible even in the light microscope, where they are seen to be organized into a series of chromomeres with large chromatin loops extended laterally. Amphibian and avian lampbrush chromosomes can be micro-surgically isolated from oocyte nucleus (germinal vesicle) with either forceps or needles In the homologous sections of the bivalent, chromatin is condensed (spirally twisted) or decondensed in the form of side loops – two per each chromosome and four at the level of the bivalent.
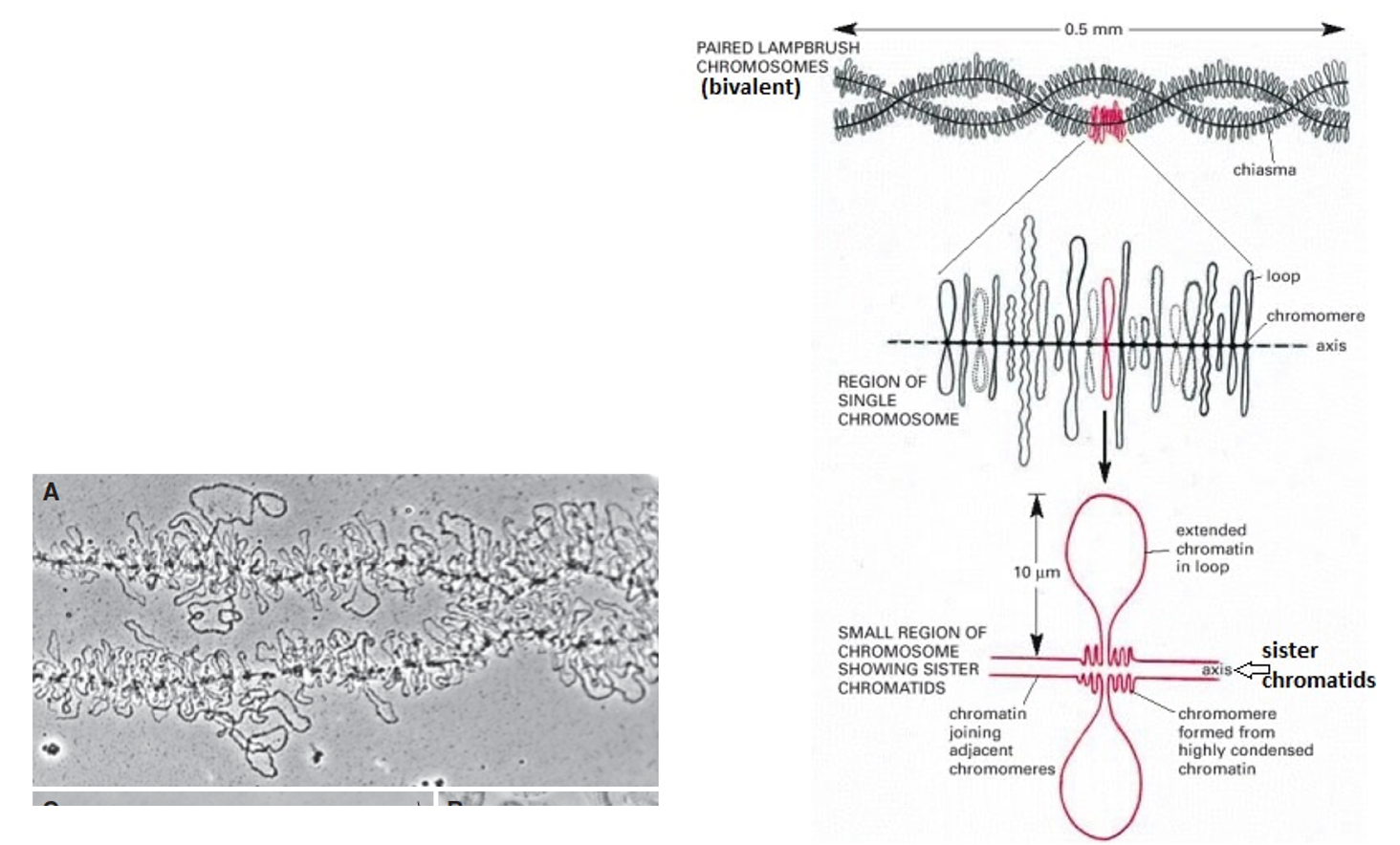
Lampbrush chromosome loops are considered an example of open chromatin. Their analogues are thought to be the “puffs” of polytenic chromosomes. They differ between each other. Polytenic chromosomes are made up of parallel chromatids, whereas lampbrush chromosome chromatin constitutes of a single DNA helix. The average length of a typical lampbrush chromosome loop is 10-15 μm, though some can be as long as50 or even 100 μm. Lampbrush chromosomes of various species have a very similar structure and perform the same function.
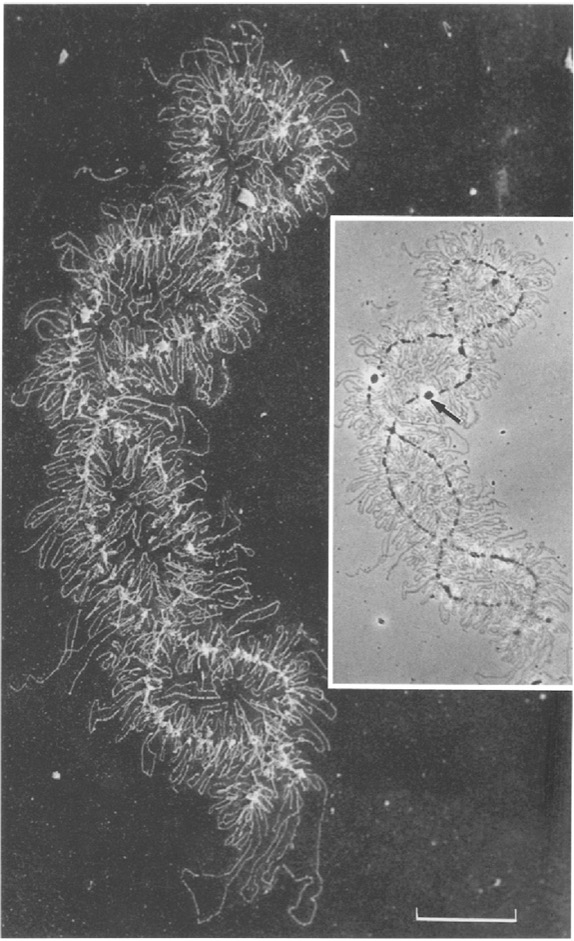
Polytene chromosomes
Polytene chromosomes are specific interphase chromosomes consisting of thousands of deoxyribonucleic acid (DNA) strands. For this reason they are very large and display a characteristic band–interband morphology. Polyteny arises in tissues, organs and at developmental stages when there is need for the rapid development of an organ at an unaltered high level of function. Organs containing cells with polytene chromosomesn are, as a rule, involved in intense secretory functions accomplished during a short time against a background of rapid growth.
Polytene chromosomes were discovered by Balbiani (1881) in larval salivary glands, Malpighian tubules, intestine, hypoderm and muscles of Chironomus plumosus as a cylindrical cord that repeatedly unrivalled and filled the nucleus. He called this structure a ‘Permanent Spireme’. Nine years later, in 1890, he found a permanent spireme in the macronucleus anlage of the infusorian Loxophyllum meleagris.
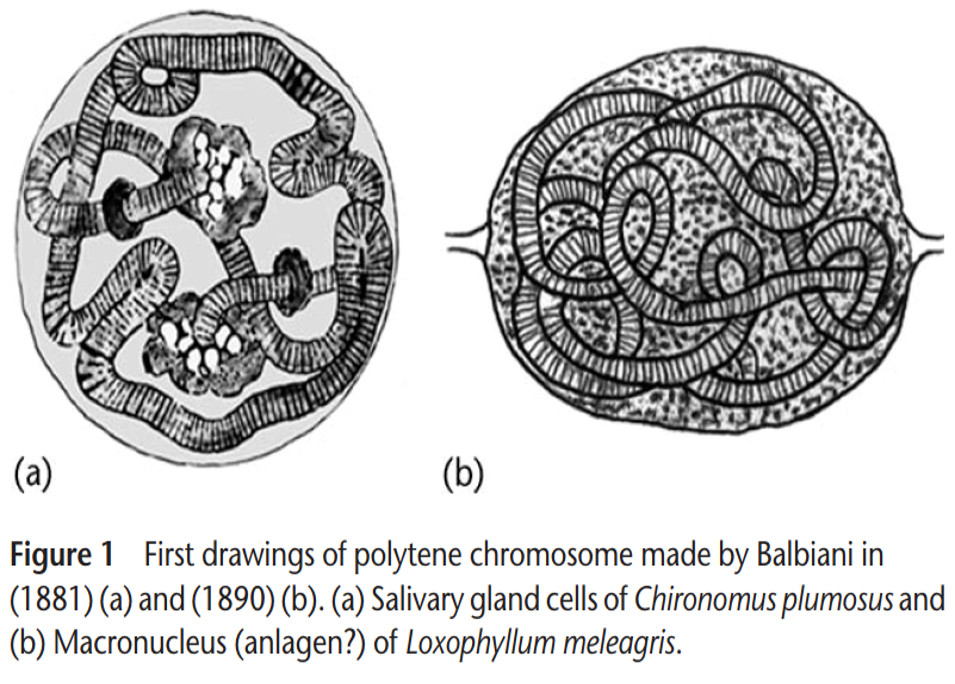
The relationship between polytene chromosomes (known as ‘giant chromosomes’ at that time) and regular mitotic chromosomes was first proposed by Rambousek (1912) and then by Kostoff (1930), Koltzoff (1934) and Bauer (1935). The giant size of the salivary gland chromosomes was explained by Koltzoff (1934) as being the consequence of multi strandedness. The term ‘Polytene’ was proposed by Koller (1935) and adopted by Darlington (1937).
In 1933–1934 scientists using squashed preparations, showed that the ‘Spireme’ is not continuous but consists of separate elements whose number is close to the haploid number of the mitotic chromosomes. Each element is formed as a result of the tight synapsis (joining together) of homologous chromosomes. Each chromosome has a definite and constant morphology and is composed of segments, displaying a distinctive transverse-banding pattern.
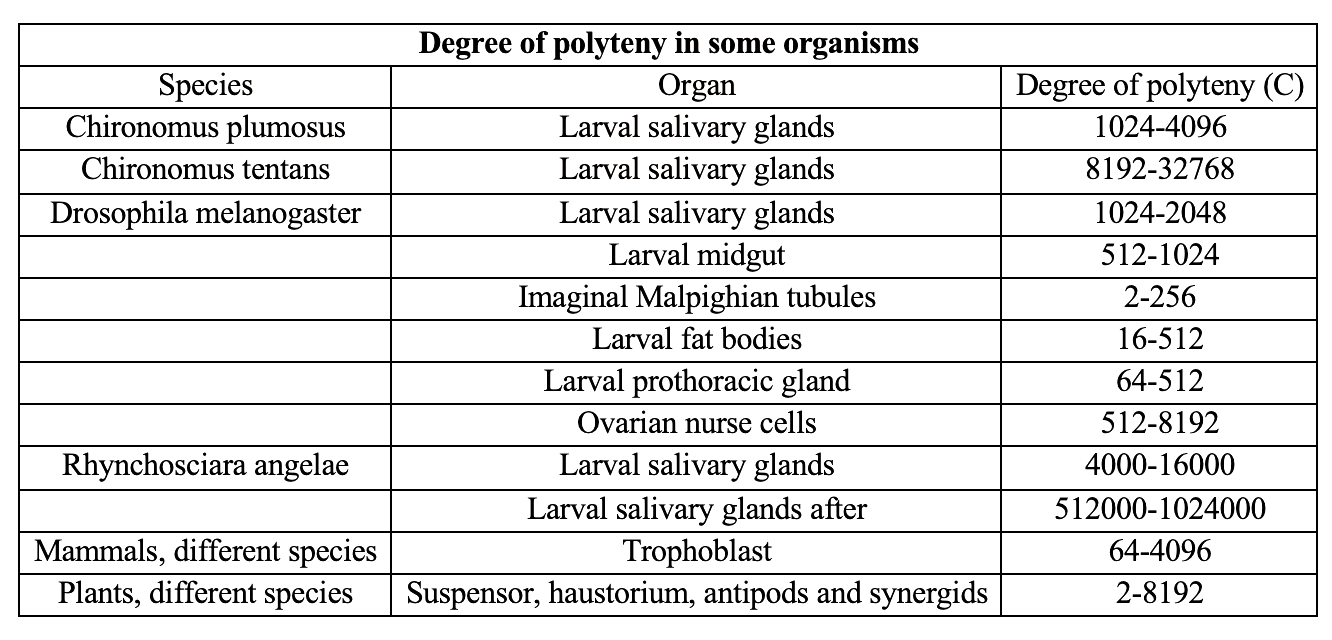
Cells with polytene chromosomes differ in the following ways from mitotically dividing cells and those undergoing endomitosis. First, the formation of polytene chromosomes is associated with the elimination of the entire mechanism of mitosis after each deoxyribonucleic acid (DNA) doubling, as a result of which the cell cycle consists of just two periods, synthetic (S) and intersynthetic (G). The polytenization cell cycle is set during midembryogenesis in Drosophila melanogaster. Second, at the end of each replication period, DNA strands do not segregate; rather, they remain paired to each other to different degrees rapid growth. The features of polyteny provide the conditions necessary to accomplish these functions. In many polytene nuclei, non homologous chromosomes are joined by their centromeric regions to form a common chromocentre. Chromocentres in polytene chromosomes correspond to heterochromatin in mitotic chromosomes. Along the linear axis of each chromatid, variation in the extent of coiling of the DNA and its associated proteins leads to variation in the concentration of the chromatin. Regions of high concentrations are known as chromomeres. For each chromatid the pattern of chromomeres is highly specific so that in the polytene chromosome homologous chromomeres align alongside each other exactly
and usually appear to fuse as a band across the polytene element.
The banding of polytene chromosome is generally such a stable and specific feature of their organization that the individual bands can be recognized, mapped and assigned reference numbers. In the interchromomeric or inter band regions, the DNA and protein concentration is lower than in the bands.
Interbands
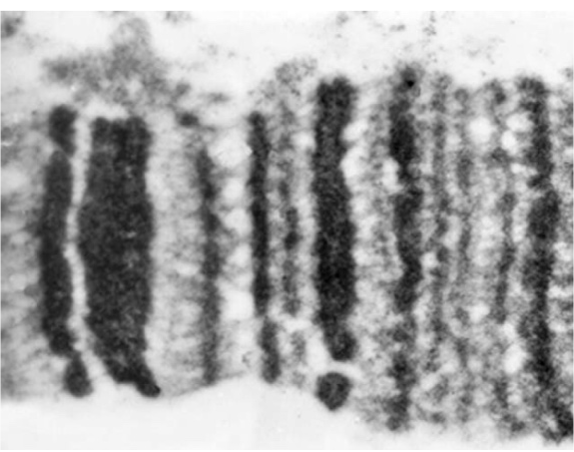